Our 1400A permeabilized fiber system is commonly used to investigate the active and passive properties of skinned, or de-membranized fibers from skeletal, cardiac muscle tissue and even some smooth muscle in some cases. The system is built around an automatic indexing chamber to help elucidate pertinent information about the myofilament, including calcium sensitivity, time to tension development and shortening velocity, among others. These demanding and complex experiments require a fast length controller and high-resolution transducer, but undesired outcomes can occur if the seemingly small details of the setup are not considered. This blog is meant to provide some helpful information to improve the quality of your measurements and increase experimental throughput.
Choosing the right microscope
Due to the nature of the experiment and size of the tissue, choosing the right microscope is imperative to success and managing throughput. Much like our previous tips and tricks blog on the 1600A system, the 1400A requires a flat-3 plate as the 802D apparatus sits directly onto the microscope and needs to lie flat and be moved in all 3 axes to properly align the fiber within the focal plane. Additionally, since the bath plate drops down to move from bath to bath and the fiber sits above the surface of the cover slip, it is imperative that the microscope has long working distance objectives with a minimum of 3mm working distance. Anything greater is a major plus. Although the scope requirements are not overly complicated, these additional 3 factors should be taken into consideration when selecting or configuring an inverted microscope.
Large stage cut out
The 802D apparatus comes with a stand for when not in use but is otherwise mounted on an inverted microscope to view the fiber from below. The design of the 802D results in a small portion of the transducer sensor head protruding below the level of the bath plate in addition to the bath plate itself moving roughly +/- 50mm when switching baths. Thus, a large plate cut out of at least 100mm diameter is required to accommodate this as shown in Figure 1.
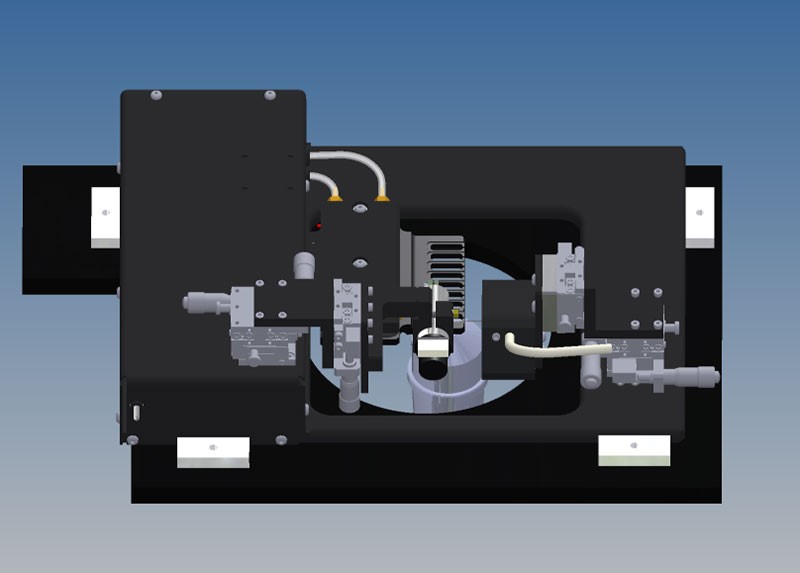
Figure 1. 802D rendering showing the large cut out of the mechanical stage below.
C-mount adapter
When it comes to single fiber mechanics, visualizing the fiber to measuring cross-sectional area, fiber length or setting sarcomere length is incredibly important. Due to its inherent striation pattern, skeletal muscle lends itself nicely to the use of our 901D HSVL sarcomere length system. To mount the camera, the inverted microscope requires a C-mount adaptor (see Figure 2). The user can then switch the port on which the light path travels to either visualize the fiber on the computer or through the eye piece.
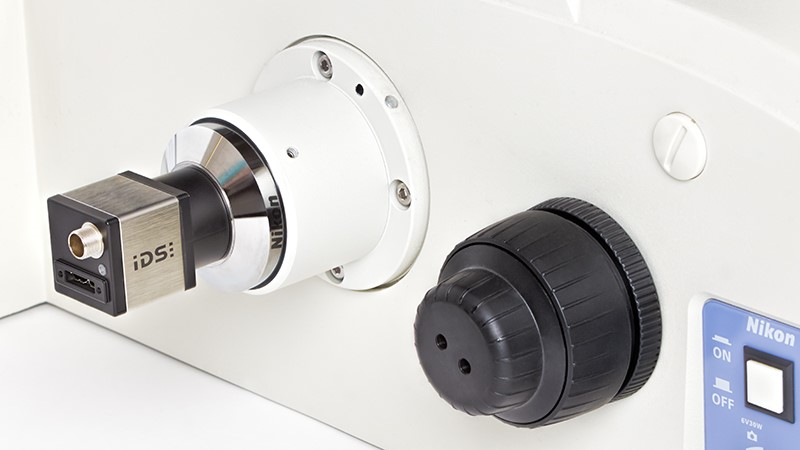
Figure 2. HSVL camera attached to the side-port C-mount adapter on an inverted microscope.
Tiltable Condenser
Although not imperative, it is incredibly useful to have microscope with a tiltable condenser. When conducting single fiber mechanics mounting is often done under a stereo microscope. This scope can either be side by side with the inverted scope (Figure 3, Left), requiring the apparatus to be lifted on and off the stage. Alternatively, the fiber can be attached under a stereoscope mounted on a boom arm (Figure 3, Right), eliminating the need to mount and dismount the apparatus from the inverted microscope. Either option lends itself to a tiltable condenser to give room for the mounting of the apparatus as well as moving the stereoscope on the boom arm in/out when required.
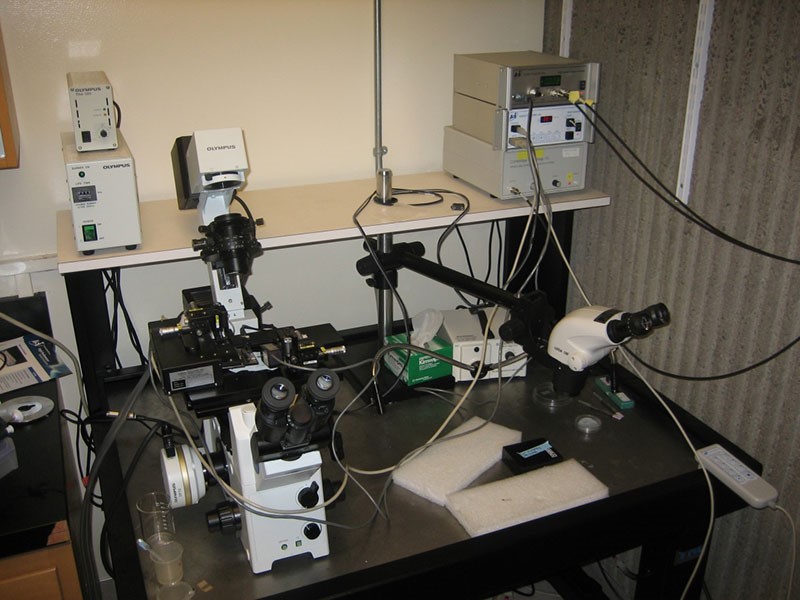
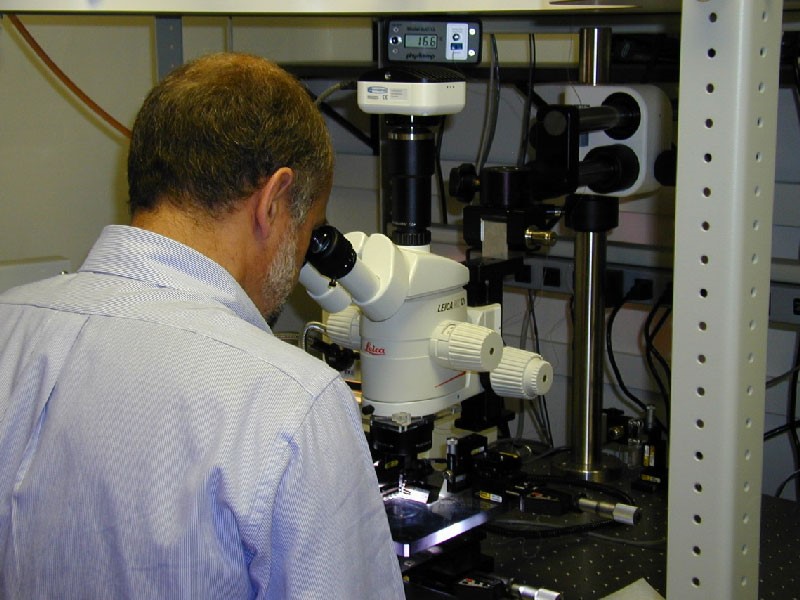
Figure 3. System arrangement showing the Stereo and Inverted Microscope Side by Side (Left) and Stereoscope mounted on a boom arm (Right).
How to mount fibers
Single fiber mechanics have been performed for many years and have contributed immensely to the understanding of the myofilament. Throughout those years, a few different ways of mounting the fibers between the motor and transducer have been developed. Here, we will outline these methods and the types of attachments required to aid you in selecting the one that suits your needs.
Shellac or Nail Polish
The first method involves the use of shellac or lacquer, or in most cases nail polish, to adhere the fiber to length controller and force transducer attachments. In this case, it is most common to use a thin gauge (28-30G) hypodermic needle or tubing as a link to the hardware. Here, you would insert a straight piece of tubing into the glass capillary tube on the transducer and bend an ‘L’ shaped piece of tubing to attach to the lever arm of your length controller (other pins options can be used; See Figure 4). Mounting the fiber to these attachments takes place in air whereby the fiber ‘sticks’ to the tubing on either side and you apply a small amount of nail polish to the ends of the fiber that are attached to the tubing, ensuring the fiber is straight and not twisted on either side.
This is allowed to dry for a couple minutes before the fiber is then submerged into the bath solution and the experimental setup can begin. Removing the fiber after measurements have been taken requires the use of acetone applied to the nail polish. This can become a bit messy, and the tubing may need to be changed periodically if the polish builds up over time. In some instances, the acetone could travel down the tubing and affect the integrity of the glue or epoxy that holds the tubing in the transducer capillary tube or onto the lever arm of the motor. In this case, extra care must be taken or a different form of glue that is resistant to acetone should be considered.
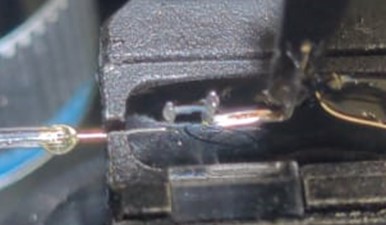
Figure 4. Muscle fiber attached to hardware using glue or in some cases nail polish. (Photo courtesy of Nishikawa Lab, NAU)
Suture
A detailed outline of tying fibers to our equipment can be found here. In short, a straight tube made from a 29G hypodermic needle can be glued to an Aurora Scientific transducer and an ‘L’ shaped tube of the same material can be bent and attached to a high-speed length controller whereby the straight posts align with one another. Using pre-looped 10-0 monofilament nylon suture, the fiber is then tied onto these posts (Figure 5).
Figure 5. Muscle fiber mounted using sutures.
T-Clips
The attachment method and likely the simplest of the three is using T-clips. These are pre-fabricated small aluminum foil clips in the shape of a ‘T’. The sides fold over and crimp onto the muscle fiber being mounted. The bottom end of the clip has a diamond shaped hole that the transducer and motor hooks slide into. These hooks are generally made into an ‘L’ shape for the transducer and a ‘J’ shape for the motor lever arm using 0.2- or 0.1-mm insect pins depending on the muscle fiber type (Figure 5). Aurora Scientific now supplies a variety of T-clips designed for specific muscle types, including skeletal, cardiac and smooth.
Figure 6. Fiber mounted to hooks using T-clips.
Experimental Setup
Once the methodology for mounting fibers has been determined it is also important to consider the experimental setup and how the mechanics measurements will be done.
Determining pre-activation strategy
Skinned or de-membranized muscle fibers lack the sarcolemma that houses calcium and thus require calcium in solution to activate the myofilament lattice and generate force. However, the uptake of calcium is diffusion limited and can occur slowly, thus determining the best pre-activation strategy is important in order to ensure the fiber is maximally activated when placed in activating solution. There are two common pre-activating strategies, the first is using a pre-activating solution whereby the fiber goes from relaxing solution (bath 1; usually 15-25C) to pre-activating solution that is weakly buffered for Ca2+ for a few minutes (bath 2; usually 15C-25C), this results in a quick activation and rapid force development once introduced to the activating solution (bath 3; usually 15-25C) (Figure 7).
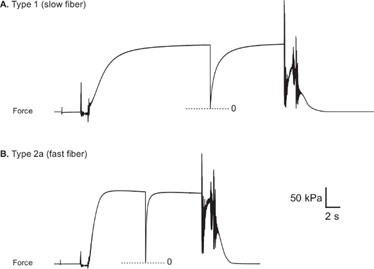
Figure 7. Representative force traces from healthy human vastus lateralis muscle fibers. (A) Type 1 fiber (B) Type 2a fiber. (Roche et al. JoVE 2015).
Figure 8. Time course of stress (force) development in a single skinned impala fibre, showing transition of the fibre through pre-activation, activation and relaxation solutions, and stress development after temperature-jump (T-jump) from 1 to 25°C. Wilson et al. Nature (2018)
The second strategy is using a jump in temperature to prime the fiber. This involves moving the fiber from cold relaxing solution (bath 1; usually 1-4C) to cold activating solution (bath 2; usually 1-4C) for a few minutes. Much like a warm pre-activating solution, this results in a rapid development of force upon bathing the fiber in warm activating solution (bath 3; usually 15-25C) (Figure 8). This method requires the use of our temperature jump variant of the apparatus (802D-120-TJ).
Setting Sarcomere Length with HVSL
As mentioned above, single fiber experiments often require a sarcomere measurement system in order to set SL of the fiber. For skeletal muscle, the natural striation patterns, work well with our High-Speed visual sarcomere length system (901D HVSL) to visualize, measure and track fiber sarcomere length. Prior to the start of experiments, it is very important to set sarcomere length to an optimal value to in order to generate maximum force. Read our blog on Why SL matters for more insight. Using HVSL, you can visualize SL and increase or decrease fiber length to reach the desired value. Unlike skeletal muscle, cardiac muscle fibers have a lattice like sarcomere pattern and thus SL is sometimes better set using a laser SL system.
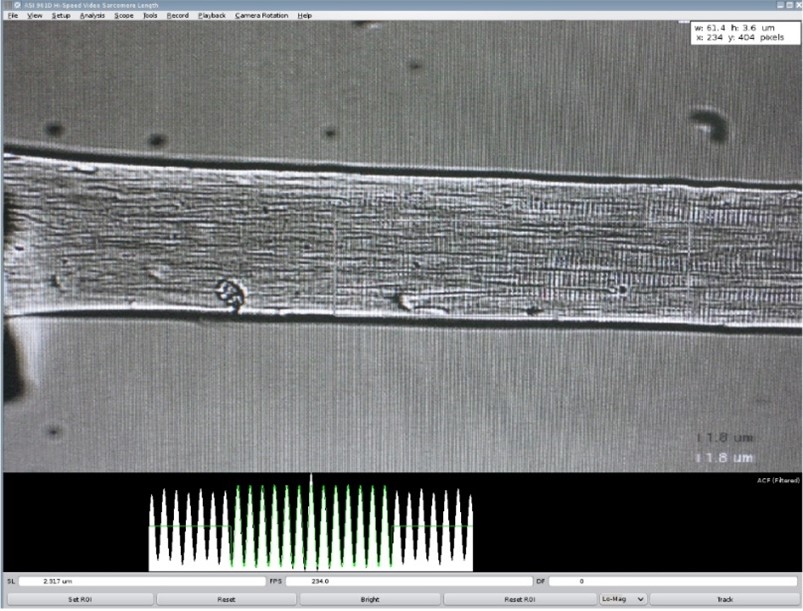
Figure 9. Single muscle fiber sarcomere striations shown in 901D HVSL.
Taking CSA measurements using prisms
Variability in fiber size is to be expected when measuring single fiber mechanics so it is important to accurately measure fiber cross-sectional area in order to calculate specific force. Specific force (sFo) is the amount of force generated (mN) per mm2 of fiber (CSA). By using CSA, you can compare the inherent capacity to generate force of each fiber, regardless of size (hypertrophy or atrophy). The 802D has two built in prisms that allow a side view of the fiber (one for inverted, one for dissecting) to properly measure fiber width in both planes as we know that the cross section of a fiber is more accurately modelled by an ellipse rather than a circle (Figure 10). Neither the prism, nor HVSL can measure the dimension of each axis of the elipse on its own. For more details, check out the JoVE article here.
Stay tuned to this blog for more upcoming tips and tricks on a wide variety of products and topics!
Figure 10. Representative fiber images illustrating each of the five corresponding diameter measurements taken in both the top and side view. (Roche et al. JoVE 2015).