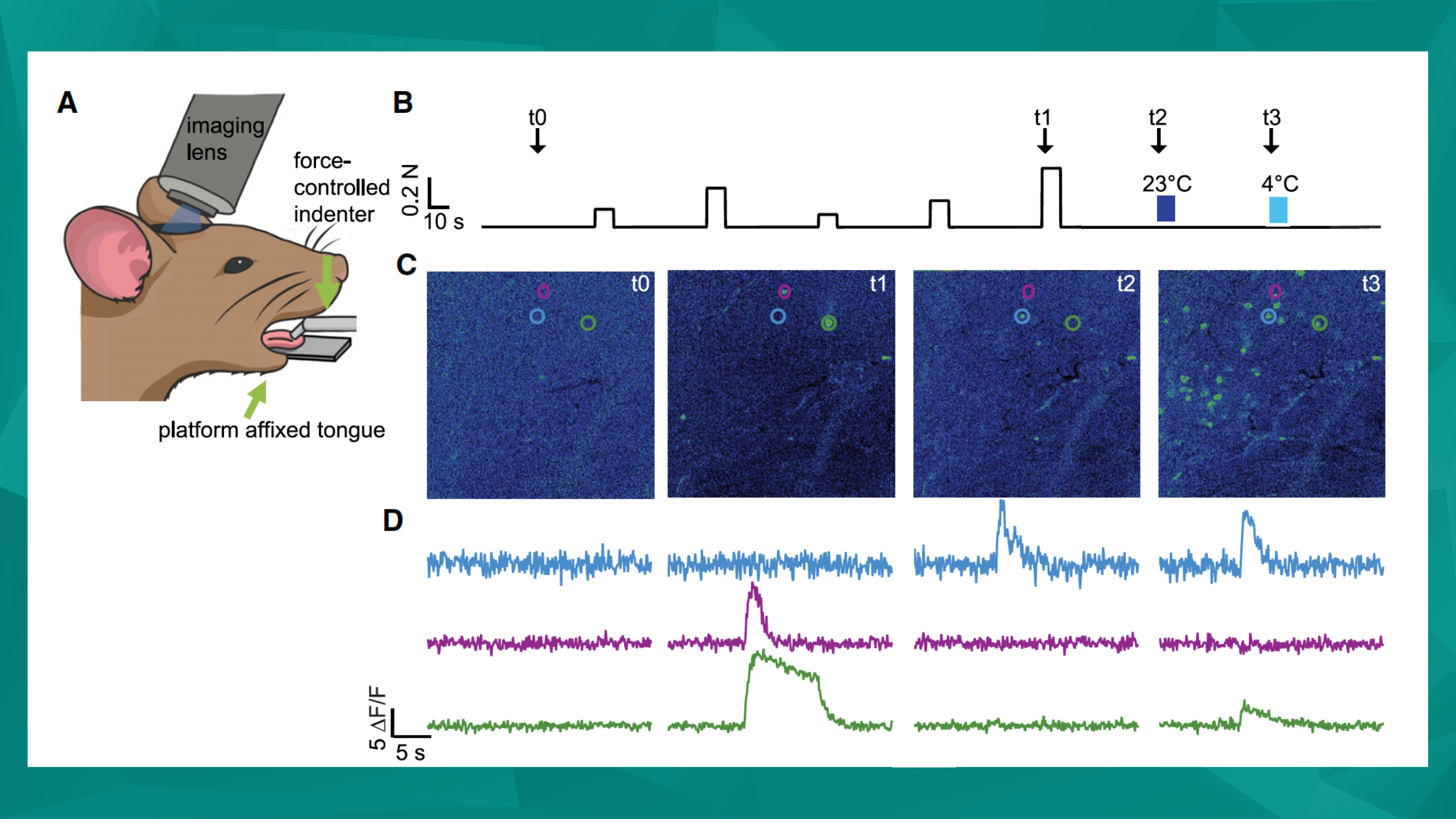
Somatosensation, the process responsible for our perception of the world, is more mechanistically complex than it may seem. Pressure, temperature, and pain are just a few of the various parameters that most organisms use to situate and navigate themselves throughout the world. While these phenomena are well described at a functional level (i.e. the five senses), this sort of communication at the cellular level is much more involved1. Nonetheless, recent publications utilizing our systems have made considerable strides in this field, unveiling some of the mechanisms and cellular basis behind mechano- and somatosensation.
Featured image (©Moayedi et al. (2023), licensed under CC BY-NC-ND 4.0), illustrates that (A) a force-controlled indenter stimulated the tongue while liquid stimuli were applied by hand to the tongue. (B) Force-controlled indention was applied to the tongue, followed by room-temperature (RT) water and then cold water. Sample image times used in (C) are indicated by arrows (t0–t3). (C) Sample images from baseline, 0.40 N indention (t1), RT water (t2), and cold water (t3). Circled cells indicate neurons responsive to cool/cold flow only (blue), pressure only (purple), and pressure and cold (green). (D) Traces from neurons in (C).
The cellular basis of mechanosensation in mammalian tongue
One of the most important yet overlooked touch-sensitive organs is the tongue, which requires sensory information to assist with feeding, speech, and social behavior. Despite its importance, little is known about the functional and molecular properties of the mechanosensory neurons within the mammalian tongue. Therefore, Moayedi et al. (2023) sought to address this by performing in vivo calcium imaging of mouse trigeminal ganglion neurons to better characterize the functional groups of mechanosensory neurons that innervate the anterior tongue. Using the 300C-I Dual-Mode Indenter with a 2 mm tip to apply mechanical sensation, pressure-evoked activity was analyzed based on temporal response dynamics.
The authors were able to distinguish five separate classes of mechanosensory neurons with distinctly different force-response relationships. Moayedi et al. (2023) also found that most lingual mechanosensory neurons were specifically tuned for dynamic stimuli – being both brush sensitive but also exhibiting transient responses to sustained pressure. Given the varied functions of the tongue, this capacity for dynamic response is not entirely unexpected, but has now been demonstrated with compelling evidence.
Properties of Nav1.8ChR2‑positive and Nav1.8ChR2‑negative afferent mechanoreceptors in the hindpaw glabrous skin of mice
While pressure is perhaps a more tangible type of mechanosensation, pain is somewhat more abstract. Thermal and mechanical pain is partially mediated by Nav1.8-positive afferent fibers, but the mechanoreceptors within these nociceptive pathways have not been fully investigated. To address this, Yamada et al. (2023) created mice expressing channel rhodopsin 2 (ChR2) in Nav1.8-positive afferents to better characterize various neural responses to mechanical stimuli within the glabrous skin of the mouse hindpaw.
In another application of the 300C-I Dual-Mode Indenter, the authors applied mechanical stimulation to the receptive field of interest to determine mechanical thresholds. Their results demonstrated that most Nav1.8ChR2negative Aβ- and Aδ-fiber mechanoreceptors are low threshold mechanoreceptors and involved in the sense of touch, whereas Nav1.8ChR2-positive Aβ-, Aδ-, and C-fiber mechanoreceptors are mainly high threshold mechanoreceptors (HTMRs) involved in mechanical perception of pain. These results have helped to further characterize nociceptive pathways and their associated mechanoreceptors.
γ-Protocadherins control synapse formation and peripheral branching of touch sensory neurons
Low-threshold mechanoreceptors (LTMR) transmit signals in response to subtle mechanical perturbations. Light touch sensation begins accordingly with the activation of LTMR endings in the skin which then propagate to the spinal cord and brainstem, but the genome level regulation of the processes controlling this body-wide intercommunication are still poorly understood. Meltzer et al. (2023) sought to address this by demonstrating that the clustered protocadherin gamma (Pcdhg) gene locus regulates somatosensory neuron synapse formation and also peripheral axonal branching.
Employing the 300C-I Dual-Mode Indenter, the authors stimulated the hindpaw as part of an in vivo spinal cord multielectrode array and electrophysiological analysis of the spinal cord. The Pcdhgc3 isoform was observed to mediate homophilic interactions between sensory axons and spinal cord neurons. Additionally, this isoform was observed to promote synapse formation in vivo and can induce postsynaptic specializations in vitro. Loss of Pcdhgs and somatosensory synaptic inputs to the dorsal horn resulted in fewer corticospinal synapses in dorsal horn neurons. The findings of Meltzer et al. demonstrate the essential role of the Pcdhg isoform in somatosensory neuron synapse formation, peripheral axon branching, and stepwise assembly of central mechanosensory circuits.
Conclusions
Recent advances in somatosensation research have shed light on the intricate mechanisms of mechanosensory systems. These studies by Moayedi et al. (2023), Yamada et al. (2023), and Meltzer et al. (2023) have provided key insights into oral mechanosensation, pain perception, and the genetic basis of sensory processing. These findings enhance our understanding of touch and pain mechanisms, highlighting the importance of appropriate measuring tools when searching for potential treatments for sensory disorders.
References:
1https://www.sciencedirect.com/science/article/abs/pii/B9780443066511500058